Nature of Heat
Heat is a form of energy contained to some extent in every substance on earth.
All known elements are made up of very small particles, known as atoms, which,
when joined together, form molecules. These molecules are particular to the
form they represent. For example, carbon and hydrogen in certain combinations
form sugar and in others form alcohol.
Molecules
are in a constant state of motion. Heat is a form of molecular
energy that results from the motion of these molecules. The
temperature of the molecules dictates to a degree the molecular
activity within a substance. For this reason, substances exist
in three different states or forms—solid, liquid, and gas. Water,
for example, may exist in any one of these states. As ice, it
is a solid; as water, it is a liquid; and as steam, it is a
gas (vapor).
When heat is added to a substance, the rate of molecular motion increases,
causing the substance to change from a solid to a liquid, and then to a gas (vapor).
For example, in a cube of ice, molecular motion is slow, but as heat is added,
molecular activity increases, changing the solid "ice" to a liquid "water".
Further application of heat forces the molecules to greater separation and
speeds up their motion so that the water changes to steam. The steam formed no
longer has a definite volume, such as a solid or liquid has, but expands and
fills whatever space is provided for it. |
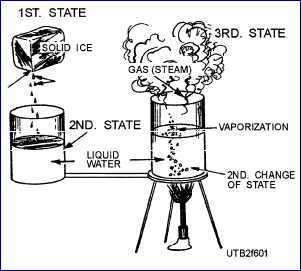
The Three States of Matter |
Heat cannot be destroyed or lost. However, it can be transferred
from one body or substance to another or to another form of
energy. Since heat is not in itself a substance, it can best
be considered in relation to its effect on substances or bodies.
When a body or substance is stated to be cold, the heat that
it contains is less concentrated or less intense than the heat
in some warmer body or substance used for comparison.
Back to Top
Units of Heat
In the theory of heat, the speed of the molecules indicates the temperature or
intensity of heat, while the number of molecules of a substance
indicates the quantity of heat.
The intensity and quantity of heat may be explained in the
following simple way. The water in a quart jar and in a 10-gallon
container may have the same intensity or temperature, but the
quantity of heat required to raise these amounts of water to
a higher uniform temperature (from their present uniform temperature)
will differ greatly. The 10 gallons of water will absorb a greater
amount of heat than the quart jar of water.
The amount of heat added to, or subtracted from, a body can
best be measured by the rise or fall in temperature of a known
weight of a substance. The standard unit of heat measure is
the amount of heat necessary to raise the temperature of 1 pound
of water 1°F at sea level when the water temperature is
between 32°F and 212°F. Conversely, it is also the amount
of heat that must be extracted to lower by 1 o F the temperature
of a pound of water between the same temperature limits. This
unit of heat is called a British thermal unit (Btu). The Btu's
equivalent in the metric system is the calorie, which is the
amount of heat required to raise one gram of water 1 o Celsius.
Suppose that the temperature of 2 pounds of water was raised
from 35°F to 165°F. To find the number of Btu required
to increase the temperature, subtract 35 from 165. This equals
a 130° temperature rise for 1 pound of water. Since 2 pounds
of water were heated, multiply 130 by 2, which equals 260 Btu
required to raise 2 pounds of water from 35°F to 165°F. Back to Top
Measurement of Heat
The usual means of measuring temperature is a thermometer. It
measures the degree or intensity of heat and usually consists
of a glass tube with a bulb at the lower portion of the tube that
contains mercury, colored alcohol, or a volatile liquid. The nature
of these liquids causes them to rise or fall uniformly in the
hollow tube with each degree in temperature change.
Thermometers are used to calibrate the controls of refrigeration.
The two most common thermometer scales are the Fahrenheit and
the Celsius.
On the Fahrenheit scale, there is a difference of 180°
between freezing (32°) and the boiling point (212°)
of water. On the Celsius scale, you have only 100° difference
between the same points (0° freezing and 100° boiling
point).
Of course, a Celsius reading can be converted to a Fahrenheit
reading, or vice versa. This can be expressed in terms of the
following formula: F = (C x 1.8) + 32 To change Fahrenheit to
a Celsius reading, the terms of the formula are as follows:
C = (F-32) ÷ 1.8 Back to Top
Transfer of Heat
Heat flows from a substance of higher temperature to bodies of
lower temperature in the same manner that water flows down a hill,
and like water, it can be raised again to a higher level so that
it may repeat its cycle.
When two substances of different temperatures are brought in
contact with each other, the heat will immediately flow from
the warmer substance to the colder substance. The greater the
difference in temperature between the two substances, the faster
the heat flow. As the temperature of the substances tends to
equalize, the flow of heat slows and stops completely when the
temperatures are equalized. This characteristic is used in refrigeration.
The heat of the air, of the lining of the refrigerator, and
of the food to be preserved is transferred to a colder substance,
called the refrigerant.
Three methods by which heat may be transferred from a warmer
substance to a colder substance are conduction, convection,
and radiation. Back to Top
Specific Heat
Specific Heat is the ratio between the quantity of heat required
to change the temperature of 1 pound of any substance 1°F,
as compared to the quantity of heat required to change 1 pound
of water 1°F. Specific heat is equal to the number of Btu
required to raise the temperature of 1 pound of a substance 1°F.
For example, the specific heat of milk is .92, which means that
92 Btu will be needed to raise 100 pounds of milk 1° F. The
specific heat of water is 1, by adoption as a standard, and specific
heat of another substance (solid, liquid, or gas) is determined
experimentally by comparing it to water. Specific heat also expresses
the heat-holding capacity of a substance compared to that of water.
A key rule to remember is that 0.5 Btu of heat is required
to raise 1 pound of ice 1° F when the temperature is below
32°F; and 0.5 Btu of heat is required to raise 1 pound of
steam 1°F above the temperature of 212°F. Back to Top
Sensible Heat
Heat that is added to, or subtracted from, a substance that changes
its temperature but not its physical state is called SENSIBLE
HEAT. It is the heat that can be indicated on a thermometer. This
is the heat human senses also can react to, at least within certain
ranges. For example, if a person put their finger into a cup of
water, the senses readily tell that person whether it is cold,
cool, tepid, hot, or very hot. Sensible heat is applied to a solid,
a liquid, or a gas/vapor as indicated on a thermometer. The term
sensible heat does not apply to the process of conversion from
one physical state to another. Back to Top
Latent Heat
Latent Heat, or hidden heat, is the term used for the heat absorbed
or given off by a substance while it is changing its physical
state. When this occurs, the heat given off or absorbed does NOT
cause a temperature change in the substance. In other words, sensible
heat is the term for heat that affects the temperature of things;
latent heat is the term for heat that affects the physical state
of things.
To understand the concept of latent heat, you must realize
that many substances may exist as solids, as liquids, or as
gases, depending primarily upon the temperatures and pressure
to which they are subjected.
To change a solid to a liquid or a liquid to a gas, ADD HEAT;
to change a gas to a liquid or a liquid to a solid, REMOVE HEAT.
Suppose you take an uncovered pan of cold water and put it over
a burner. The sensible heat of the water increases and so does
the temperature. As you continue adding heat to the water in
the pan, the temperature of the water continues to rise until
it reaches 212°F. What is happening? The water is now absorbing
its latent heat and is changing from a liquid to a vapor. The
heat required to change a liquid to a gas (or, the heat that
must be removed from a gas to condense it to a liquid) without
any change in temperature is known as the LATENT HEAT OF VAPORIZATION.
Now
suppose you take another pan of cold water and put it in a place
where the temperature is below 32°F. The water gradually
loses heat to its surroundings, and the temperature of the water
drops to 32°F until all the water has changed to ice. While
the water is changing to ice, however, it is still losing heat
to its surroundings. The heat that must be removed from a substance
to change it from a liquid to a solid (or, the heat which must
be added to a solid to change it to a liquid) without change
in temperature is called the LATENT HEAT OF FUSION. Note the
amount of heat required to cause a change of state (or the amount
of heat given off when a substance changes its state) varies
according to the pressure under which the process takes place.
The
picture shows the relationship between sensible heat and latent
heat for one substance – water at atmospheric pressure.
To raise the temperature of 1 pound of ice from 0°F to 32°F,
you must add 16 Btu. To change the pound of ice at 32°F
to a pound of water at 32°F, you add 144 Btu (latent heat
of fusion). There is no change in temperature while the ice
is melting. After the ice is melted, however, the temperature
of the water is raised when more heat is applied. When 180 Btu
are added, the water boils. To change a pound of water at 212°F
to a pound of steam at 212°F, you must add 970 Btu (latent
heat of vaporization). After the water is converted to steam
at 212°F, the application of additional heat causes a rise
in the temperature of the steam. When you add 44 Btu to the
steam at 212°F, the steam is superheated to 300°F. Back to Top
Total Heat
Total Heat is the sum of sensible heat and latent heat. Since
measurements of the total heat in a certain weight of a substance
cannot be started at absolute zero, a temperature is adopted at
which it is assumed that there is no heat; and tables of data
are constructed on that basis for practical use. Data tables giving
the heat content of the most commonly used refrigerants start
at 40°F below zero as the assumed point of no heat; tables
for water and steam start at 32°F above zero. Tables of data
usually contain a notation showing the starting point for heat
content measurement.
Back to Top
Day-Ton of Refrigeration
A day-ton of refrigeration (sometimes incorrectly called a ton
of refrigeration) is the amount of refrigeration produced by melting
1 ton of ice at a temperature of 32°F in 24 hours. A day-ton
is often used to express the amount of cooling produced by a refrigerator
or air-conditioner. For example, a 1-ton air-conditioner can remove
as much heat in 24 hours as 1 ton of 32°F ice that melts and
becomes water at 32°F.
It is a rate of removing heat, rather than a quantity of heat.
A rate can be converted to Btu per day, hour, or minute. To
find the rate, proceed as follows:
Per Day: Multiply 2,000 (number of pounds of ice in 1 ton)
by 144 (latent heat of fusion per pound) = 288,000 Btu per day
Per Hour: 288,000 (Btu per day) ÷ 24 (hours in a day)
= 12,000 So, a "1-ton" air-conditioner would have
a rating of 12,000 Btu per hour. Back to Top
Pressure
Pressure is defined as a force per unit area. It is usually measured
in pounds per square inch (psi). Pressure may be in one direction,
several directions, or in all directions, as shown in picture
below. The ice (solid) exerts pressure downward. The water (fluid)
exerts pressure on all wetted surfaces of the container. Gases
exert pressure on al I inside surfaces of their containers.
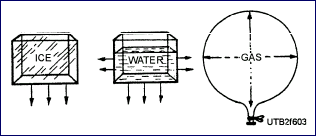
Exertion of Pressure
Pressure is usually measured on gauges that have one of two
different scales. One scale is read as so many pounds per square
inch gauge (psig) and indicates the pressure above atmospheric
pressure surrounding the gauge. The other type of scale is read
as so many pounds per square inch absolute (psia) and indicates
the pressure above absolute zero pressure (a perfect vacuum).
Atmospheric Pressure
Atmospheric pressure is the pressure of the weight of air above
a point on, above, or under the earth. At sea level, ATMOSPHERIC
PRESSURE is 14.7 psia, as shown in picture below. As one ascends,
the atmospheric pressure decreases about 1.0 psi for every 2,343
feet. Below sea level in excavations and depressions, atmospheric
pressure increases. Pressures under water differ from those
under air only because the weight of the water must be added
to the pressure of the air.
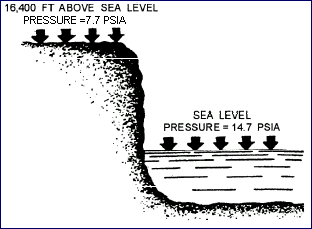
Atmospheric Pressure
Scale Relationships
A relationship exists between the readings of a gauge calibrated
in psig and calibrated in psia. As shown in the picture below,
when the psig gauge reads 0, the psia gauge reads the atmospheric
pressure (14.7 psia at sea level). In other words, the psia
reading equals the psig reading plus the atmospheric pressure
(7.7 psia at 16,400 feet), or, a psig reading equals the psia
reading minus the atmospheric pressure.
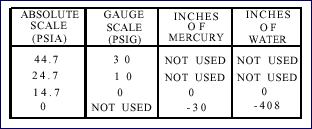
Scale Relationships
For pressure less than the atmospheric pressure (partial vacuums),
a measuring device with a scale reading in inches of mercury (Hg)
or in inches of water (H2O) is used. A perfect vacuum is equal
to -30 inches of mercury or -408 inches of water (fig. 6-5). In
refrigeration work, pressures above atmospheric are measured in
pounds per square inch, and pressures below atmospheric are measured
in inches of mercury.
Effects of Pressure on Gases
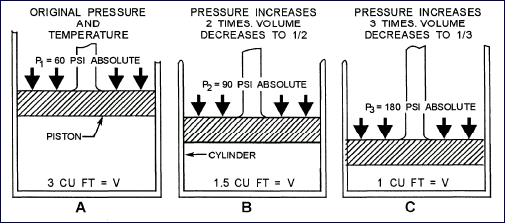
Pressure-Volume Relationship
The exertion of pressure on a substance with a constant temperature
decreases its volume in proportion to the increase of pressure.
For example, suppose that a given amount of gas is placed in
a cylinder that is sealed on one end and has a movable piston
on the other end. When 60 psi of absolute pressure is exerted
on the piston, as shown in view A of picture above, the volume
of the gas is compressed to 3 cubic feet. When 90 psi of absolute
pressure is exerted on the piston, as shown in view B, the volume
of the gas is compressed to 1.5 cubic feet. Finally, when 180
psi of absolute pressure is exerted on the piston, as shown
in view C, the volume of the gas is compressed to 1 cubic foot.
Thus, if a given amount of gas is confined in a container and
subject to changes of pressure, its volume changes, so the product
of volume multiplied by absolute pressure is always the same.
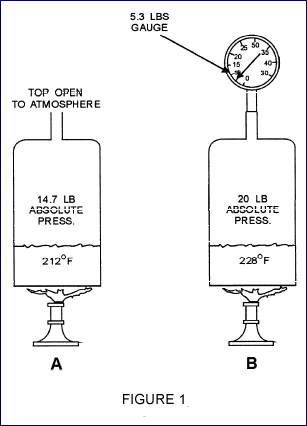 |
Pressure has a relationship to the boiling
point of a substance There is a definite temperature at
which a liquid boils for every definite pressure exerted
upon it. For instance, water boils at 212°F at atmospheric
pressure (14.7 psia), shown in view A, figure 1.
The same water boils at 228°F if the pressure is raised
5.3 psig (20 psia), as shown in view B, figure 1.
This effect of reduced pressure on the boiling temperature
of refrigerants makes the operation of a refrigeration
system possible. The pressure temperature relationship
chart below gives the pressures for several different
refrigerants.
An increase in the temperature of a refrigerant results
in an increase in pressure, and a decrease in temperature
causes a decrease in pressure. By the same token, a
decrease in pressure results in a corresponding decrease
in temperature.
This means that as the pressure of a refrigerant is
increased, so is the temperature at which the refrigerant
boils. Thus, by regulating the pressure of the refrigerant,
the temperature at which evaporation takes place and
at which the latent heat of evaporation is used can
be controlled. |
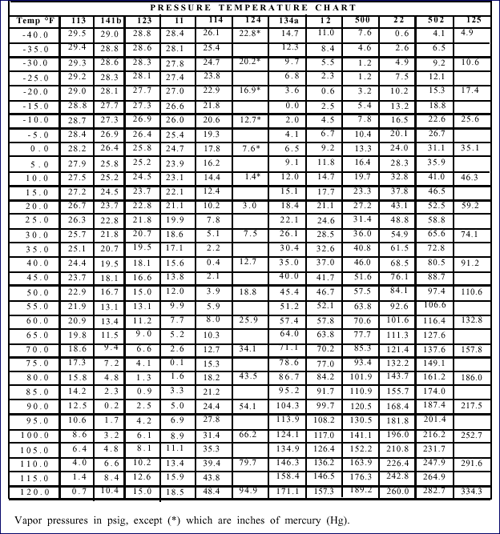
Pressure Temperature Chart
Back to Top
Vaporization
Vaporization is the process of changing a liquid to vapor,
either by evaporation or boiling. When a glass is filled with
water, as shown in picture above, and exposed to the rays of
the sun for a day or two, you should note that the water level
drops gradually. The loss of water is due to evaporation. Evaporation,
in this case, takes place only at the surface of the liquid.
It is gradual, but the evaporation of the water can be speeded
up if additional heat is applied to it. In this case, the boiling
of the water takes place throughout the interior of the liquid.
Thus the absorption of heat by a liquid causes it to boil and
evaporate.
Vaporization can also be increased by reducing the pressure
on the liquid, as shown above
Pressure reduction lowers the temperature at which liquid boils and hastens its evaporation.
When a liquid evaporates, it absorbs heat from warmer surrounding objects and cools them.
Refrigeration by evaporation is based on this method. The liquid is allowed to expand under
reduced pressure, vaporizing and extracting heat from the container (freezing compartment),
as it changes from a liquid to a gas.
After the gas is expanded (and heated), it is compressed, cooled, and condensed into a liquid again.
Back to Top
Condensation
Condensation is the process of changing a vapor into a liquid.
For example, a warm atmosphere gives up heat to a cold glass
of water, causing moisture to condense out of the air and
form on the outside surface of the glass. Thus the removal
of heat from a vapor causes the vapor to condense.
An increase in pressure on a confined vapor also causes
the vapor to change to a liquid. This fact to the right.
When the compressor increases the pressure on the vapor,
the condensing vapor changes to a liquid and gives up
heat to the cooler surrounding objects and atmosphere.
These conditions exist when the vaporized refrigerant
is compressed by the compressor of a refrigeration system
and forced into the condenser. The condenser removes the
superheat, latent heat of vaporization, and, in some cases,
sensible heat from the refrigerant. |
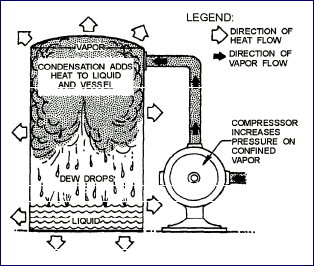 |
Back to Top
|